Introduction
Dynamic knee valgus (DKV) is a significant risk factor for several lower limb injuries [
1,
2]. In specific, DKV has been identified as a modifiable risk factor for anterior cruciate ligament (ACL) injuries [
3, 4] and patellofemoral pain syndrome [
5, 6]. Athletes with DKV perform sport-specific tasks with the femur in adduction and internal rotation, the tibia in abduction, anterior translation, and internal rotation, and the ankle in an inversion position [
1]. According to previous studies, female athletes have a higher probability than male athletes to land with DKV, increasing the risk of ACL injury [
7, 8]. DKV can result from various factors, including but not limited to inadequate hip neuromuscular control, increased femoral and tibial torsion, a broader pelvic, and increased midfoot movement [
9].
To understand the possible mechanisms of increased injuries among athletes with DKV, several studies were conducted to analyze the biomechanics of different functional tasks [
10-
14]. For instance, previous studies showed that people with DKV exhibited improper movement patterns with a medial knee position, distal tibial abduction, or valgus knee excursion during sport-related tasks [
10,
13, 14]. Moreover, it has been demonstrated that healthy subjects with a dynamic valgus alignment showed more lateral compartment stress [
12]and different frontal plane kinematics and kinetics during gait [
13, 14].
Walking is a dynamic activity that requires exquisite neuromuscular control that works in coordination with proprioceptive, vestibular, and visual sensors to generate coordinated limb movement [
15]. Human gait is a complicated task that requires multi-joint coordination to move smoothly and maintain balance [
15]. Inter-joint coordination refers to how the movements of two or more joints are linked together, which highlights the importance of sequencing and precise timing of neuromuscular system control over the degrees of freedom in the biomechanical system. This coordination is critical to achieve smooth and effective movement, as it allows all the joints to work together in a coordinated and synchronized manner [
16]. The nervous system must adapt to alterations in the control strategies, such as intra-limb coordination, to preserve the effectiveness and smoothness of walking [
17, 18].
Coordination is an essential concept in motor control and biomechanics [
19]. Coordination is a nonlinear method of analysis of dynamics that measures the between segments magnitude of motion and relative timing [
20, 21]. Examining the relative phase relationship may also provide quantitative information regarding to how joint segments are coordinated during movement [
20]. In contrast, the coordination variability of joint couplings has a crucial role in the formation of optimal movement patterns, opposite to the conventional belief that all movement variability is undesired [
21-
23].
As mentioned above, DKV is a common musculoskeletal deviation among female athletes [
24]. Moreover, previous studies showed that athletes with DKV are prone to experience lower extremity injuries [
1,
24]. Therefore, several studies were conducted to find potential explanations regarding to biomechanical changes underlying musculoskeletal injuries among athletes with DKV [
21-
23]. On the other hand, it has been suggested that coordination is an essential concept in the investigation of human movement [
20, 21]. In addition, no research has been conducted to investigate the coordination patterns of the lower extremities during walking in athletes with DKV; therefore, this study was conducted to investigate lower limb coordination during walking in athletes with and without DKV.
Materials and Methods
This case-control study included 32 recreational female athletes who volunteered to participate in the research. The athletes were allocated to two groups, 17 participants with DKV and 15 participants without DKV (WDKV). The convenience sampling method was utilized to recruit participants. The inclusion criteria included women aged 18-30 years who had consistent physical activity at least three sessions/weeks for the previous three years. The DKV group was determined based on the presence of significant knee flexion during single-leg squats (SLS). The exclusion criteria included having any medical disorder that limits activity, inability to perform the test, previous internal surgeries, lower back pain at the time of testing, transfer or loss of training for at least 3 days in the last six months due to internal injuries, and physical fitness, a critical factor according to the New York posture rating. The Biomedical Research Ethics Board of Allameh Tabataba’i University approved the research methods before starting the study, and all participants gave written informed consent. All participants were wearing sportswear and were barefoot during the test.
In this study, the dominant leg was considered as the one that was used to kick the ball in at least two of three shots. The dominant leg data was analyzed as research data. The SLS test is used to identify athletes with DKV. The athletes were barefoot and requested to stand on one leg and bend their knees to 90° while placing their hands on their hips. The athletes were requested to squat down with their knees at a 30° angle and hold this position for a while before returning to their knees. Videos of the SLS performances were created and analyzed using Kinovea software, version 0.8.27 to identify the 30° of knee flexion angle. Otherwise, the subject was verbally cued to adjust their knee flexion during subsequent squats. Each athlete was asked to repeat the SLS test three times on each leg, and the examiners recorded any abnormal movements, such as the Trendelenburg sign, swinging of the arms, or valgus collapse of the supported knee. The SLS test was considered positive if more than two aberrant motions were observed with the main weight-bearing leg during the test. All participants scored on the SLS test; positive results indicated low-grade muscle weakness, weak core, or weakness of the hip abductors. Two examiners with more than 3 years of training and experience in the assessment and rehabilitation of the neuromusculoskeletal system reviewed the SLS results.
Data collection
A motion analysis system with an eight-camera (from Motion Analysis Corporation, based in Santa Rosa, USA) was used to gather kinematic data at 200 Hz. A total of 24 markers with a diameter of 19 mm that reflect infrared light were attached to specific bony landmarks. The landmarks were selected according to visual 3D guidelines and included the anterior superior iliac spine, posterior superior iliac spine, iliac crest, medial and lateral malleolus, lateral and medial femoral condyles, heels, fifth, second, and first metatarsal heads, and C7 and T10 vertebrae (
Figure 1).
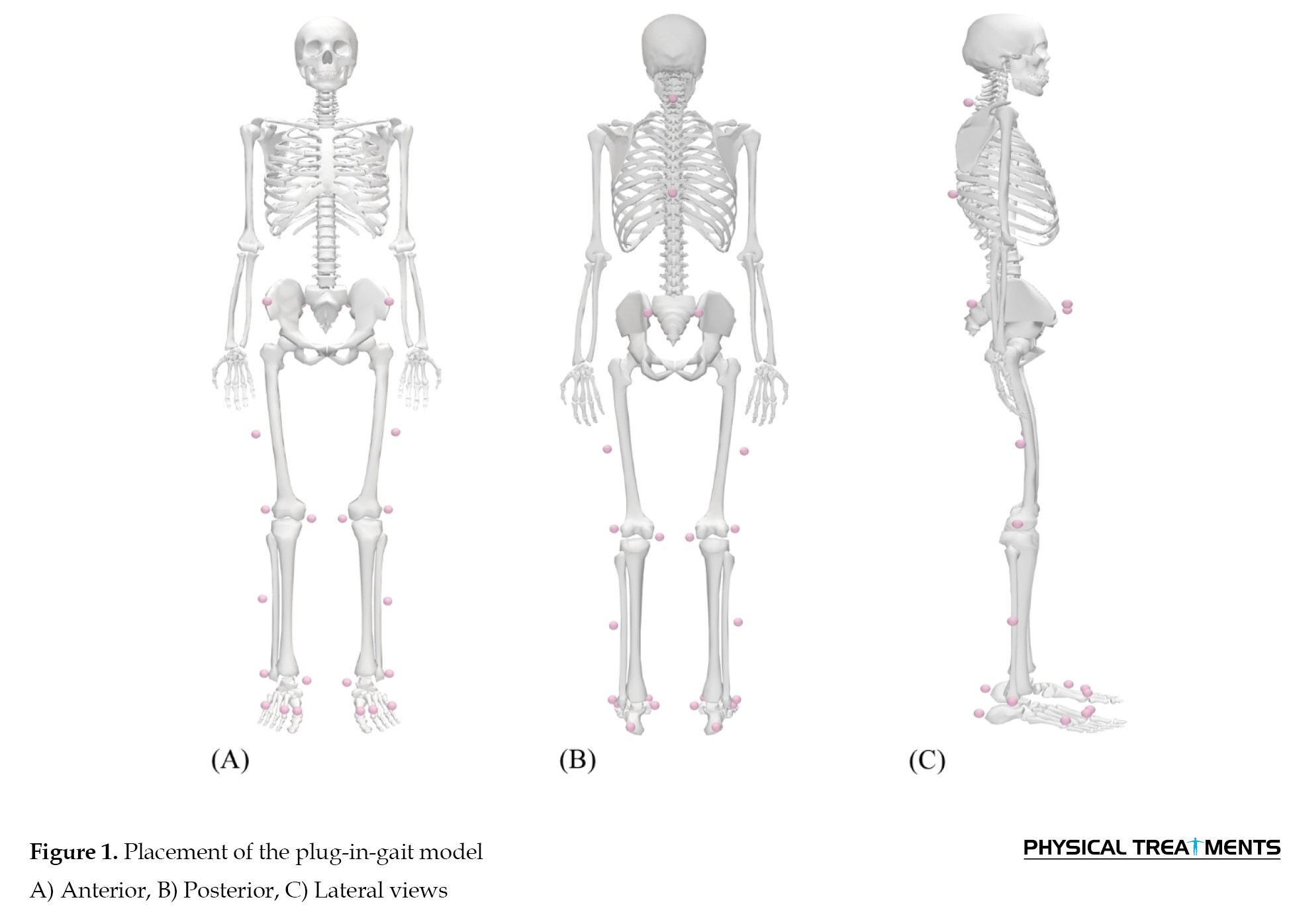
The warm-up before the test was considered 10 minutes. Before the gait trials, a static standing trial was recorded to develop a model. The participants started moving upon hearing the command “run” from the examiner while focusing on a fixed point at eye level at the end of the path to induce a natural gait pattern. The study participants were instructed to walk unshod on an 8-meter pathway at a comfortable pace that mimicked their daily walking style. To ensure consistent gait initiation, a starting point was determined that allowed each participant to complete four full gait cycles before touching the force platforms to stabilize their gait speed. The participants were not under other restrictions, and researchers collected at least fifteen walking passes at a comfortable pace to obtain enough data for analysis. A ten-second break was given between trials. If a participant’s gait behavior was deemed insufficiently similar to their daily walking style, that trial was discarded, and another trial was performed without notifying the participant. Valid trials were defined as those where all markers were visible and identifiable, and ten such valid gait cycles were chosen for further analysis.
The collected raw data were filtered using a low-pass, fourth-order Butterworth filter with a cutoff frequency of 6 Hz through the Visual3D motion analysis program (C-Motion, Rockville, MD, USA). The collected trajectory data was filled in if necessary to account for any gaps. These gaps were usually very small, typically only 2-3 frames wide, and in sporadic cases, up to 10 frames wide. The gap-filling was done using the Visual3D software. An eight-component model of the body was used, including the pelvis, right and left thighs, shanks, and feet, all of which were stiff. The segmental angles in each motion plane were then calculated using the MATLAB software, version 9.14.
Inter-segmental coordination analysis
The high-pass algorithm technique detected the heel-strike and toe-off events. This technique is known for its precision and accuracy in recognizing events in both normal and pathological gaits [
25]. In addition, the researchers visually examined the identified events to ensure that no events were incorrectly detected due to missing marker data. The continuous relative phase (CRP) method was used to evaluate inter-segmental coordination. A biomechanics expert wrote MATLAB software code to analyze the biomechanical data. Each segmented time series was interpolated to 100% of the cycle on each path using the cubic spline function. An experimental mode decomposition algorithm was used to determine the assumptions to be plotted and the motor usage of the sinusoidal signal. All empirical modes, except the lowest frequencies, were used to reconstruct the time series [
26]. The segments’ angular velocity in three cordinal planes of motion were obtained from the calculated segment angles using a first central difference approach [
27].
Phase portraits of every segment were estimated to examine the CRP, by plotting the segment’s normalized angular position (θ [i.norm]) on the x-axis against the segment’s normalized angular velocity (ω_[i.norm]) on the y-axis. The data were normalized to reduce individual variances and lessen the effects of changes in movement frequencies and amplitudes [
26]. The angular positions were normalized using an equation so that they were within a range of -1 to 1, with zero at the center (as shown in
Equation 1) [
28]. These phase portraits were then used to investigate the CRP.

During a complete gait cycle, θi represents the angular velocity of all 100 interpolated data points.
The angular velocity was normalized based on the maximum absolute velocity throughout the motion using
Equation 2, and zero represented zero velocity [
28]:
2. ωi.norm=ωi⁄(max(|ωi|),i=1…100)
In a full gait cycle ωi, represents the angular velocity of all 100 interpolated data points.
Next, for each data point, the phase angles (φ) throughout the entire gait cycle were computed using the arctangent function, as shown in
Equation 3 [
28]:
3. φi=tan-1 (ωi.norm⁄θi.norm)
Simply put, the angle is drawn by the positive X-axis of the phase plot, and the line connecting the origin to each data point forms the phase angle (
Figure 2).
The subsequent step involved computing the CRP, which demonstrates how two interacting segments are linked throughout the entire performance of a task. By taking the absolute value of the difference between the distal and proximal segment phase angles, the CRP achieved at each point during the gait swing and stance phases, as shown in the following
Equation 4:
4. CRPi=|φi.Pj-φi.Dj|
In the equation, the phase angle of the proximal and distal joints is represented by φPj and φDj respectively
(φPelvis-Thigh, φ (Thigh-Shank ), φShank-Foot)
CRP values close to zero indicate more synchronized motion, demonstrating that the segments are moving in the same direction. Conversely, CRP values closer to 180 suggest more out-of-sync motion, meaning that the segments move in opposite directions [
29]. The discontinuity at 0.360 was corrected by subtracting numbers greater than 180 from 360 [
27]. For each connection segment, the CRP curve was averaged over 10 cycles, and the mean average curve was calculated for each participant. From the full curve, two additional parameters were generated, which were quantified once and tested for differences.
The first indicator is the mean absolute relative phase (MARP), which is calculated by taking the mean value of all points of the curve in a period (rest phase and swing phase) as shown in
Equation 5:
5. MARP=∑P i=1|CRP|i⁄P
In the equation, the number of data points during each phase of the analysis is represented by P. A low and high MARP values show more synchronization and out-of-phase oscillation between the segments, respectively [
30].
The second parameter, deviation phase (DP), was used to quantify the coordination variability. It is calculated by taking the standard deviation points of all CRP curve points during the swing and stance phases of the walking cycle (representing different cycles), respectively, shown in
Equation 6:
6. DP= ∑P i=1SDi⁄p
In the equation, in each phase, the number of data points is represented by p, and the standard deviation for each data point is represented by [SD]i. A small DP value indicates that the link between the two segment movements is less likely to vary or that a more consistent pattern of inter-segment coordination across trials exists [
30].
The means for both parameters and segmental relationships during the swing and stance phases were determined for the group.
Statistical analysis
The collected data was analyzed using SPSS software, version 24. The normal distribution of the data was tested using the Shapiro-Wilk test. The means of DP and MARP values during the stance and swing phases of gait were compared between two groups using the independent samples t-test. Throughout this study, a significance level of 0.05 was considered. Additionally, the effect size (ES) was used to show the clinical significance of mean changes.
Results
Demographic properties of the participants
Table 1 presents the demographic data for the participants. No significant between-group difference was in the following parameters, height, body mass, age, and body mass index (BMI).
Pelvis-thigh coordination
The analysis of pelvis-thigh coordination in cardinal planes during walking gait showed significant differences in mean DP MARP values between the two groups (P<0.05). Specifically, the means of DP in the stance (frontal [P<0.001], sagittal [P=0.021], and transverse [P<0.001] planes) and swing phases (frontal [P<0.001], sagittal [P<0.001], and transverse [P<0.001] planes), as well as the means of pair MARP in the stance phase (frontal [P<0.001] and transverse [P<0.001] planes), and swing phase (transverse [P<0.001] plane) showed statistically substantial differences.
Figure 3 and
Table 2 present these results.
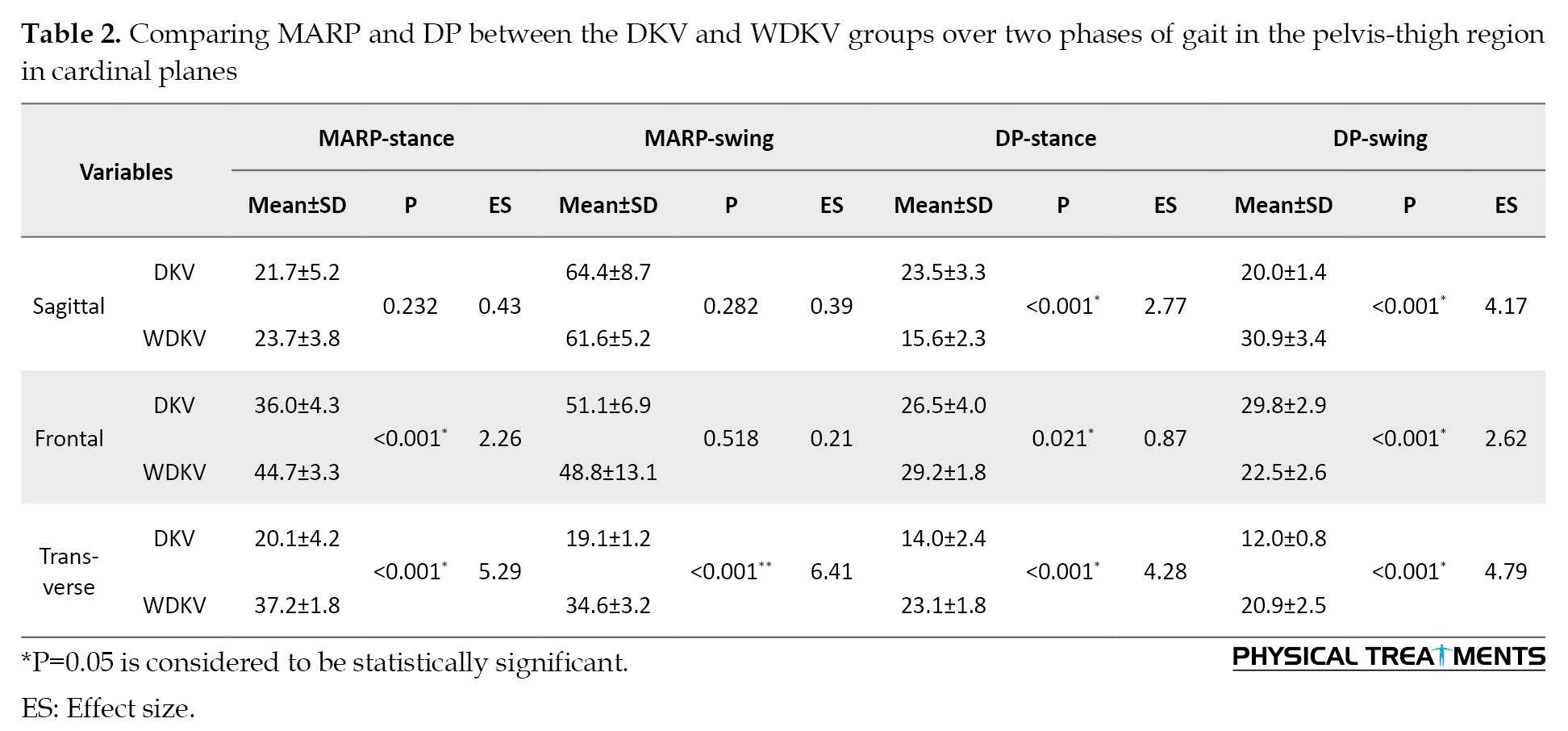
Thigh-shank coordination
The study analyzed thigh-shank coordination in cardinal planes during gait and found significant differences in DP and MARP values between the two groups (P<0.05). Specifically, the means of DP in the stance (frontal [P<0.001] and transverse [P<0.001] planes) and swing phases (frontal [P<0.001] and transverse [P<0.001] planes), as well as the means of pair MARP in the stance phase (saggital [P<0.001], frontal [P<0.001], and transverse [P<0.001] planes), and swing phase (saggital [P<0.001], frontal [P<0.001], and transverse [P<0.001] planes) showed significant differences.
Figure 4 and
Table 3 present these results.
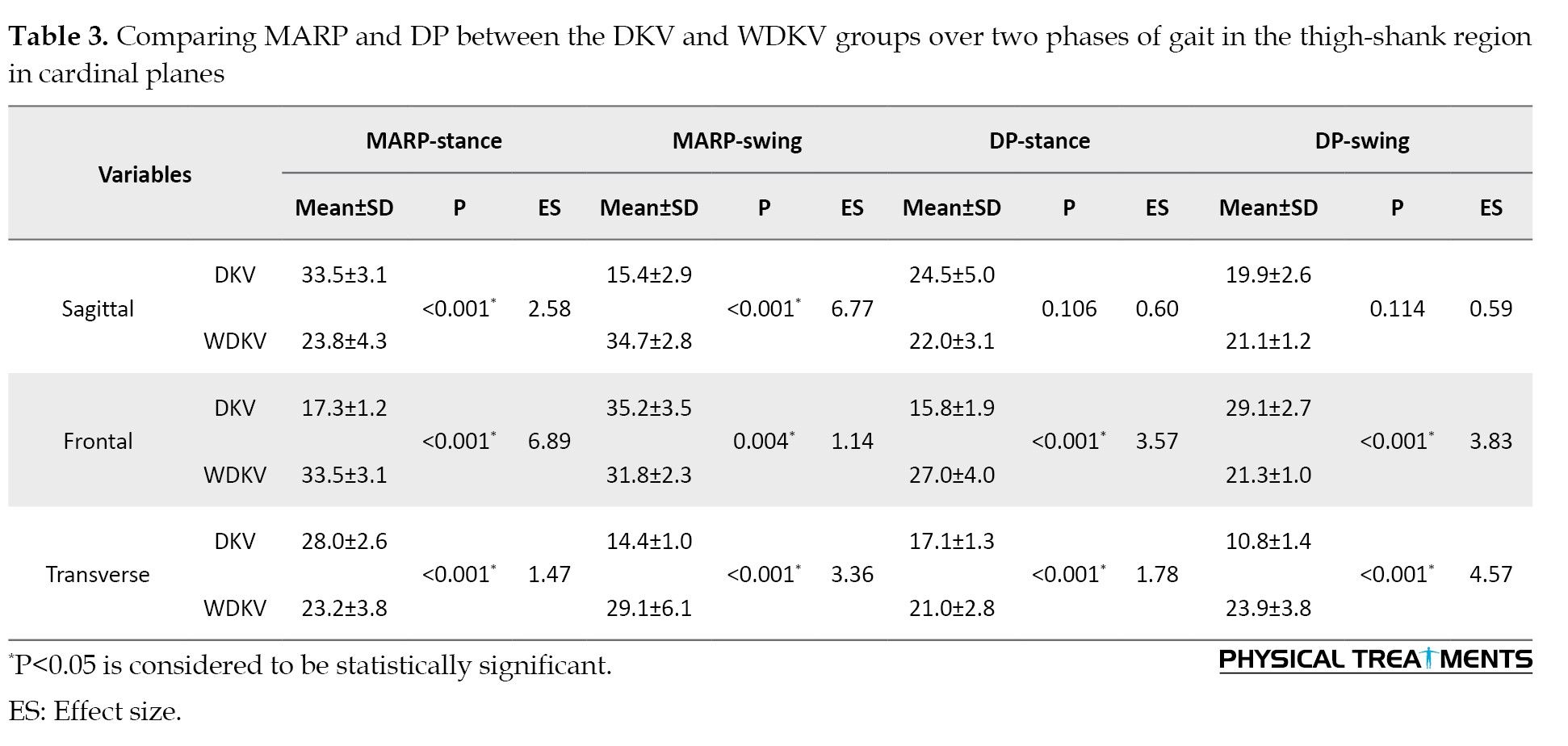
Shank-foot coordination
The study analyzed thigh-shank coordination in the cardinal planes during gait and found significant differences in DP and MARP values between the two groups (P<0.05). Specifically, the means of DP in the stance (saggital [P<0.001], frontal [P<0.001], and transverse [P<0.001] planes) and swing phases (saggital [P=0.040], frontal [P<0.001], and transverse [P<0.001] planes), as well as the means of pair MARP in the stance phase (saggital [P<0.001], frontal [P<0.001], and transverse [P<0.001] planes), and swing phase (saggital [P<0.001], frontal [P<0.001], and transverse [P<0.001] planes) showed significant differences.
Figure 5 and
Table 4 present these results.
Discussion
This study discovered that female athletes with DKV displayed distinct intersegmental coordination patterns between the thigh-shank, pelvis-thigh, and shank-foot in their lower extremities during walking, compared to athletes without DKV.
Female athletes with DKV display distinct pelvic-thigh coordination patterns in both phases of walking. The current results are consistent with other literature, showing that DKV is associated with biomechanical changes in athletes [
24,
31,
32]. In the stance phase, they show a significantly greater mean DP in the sagittal plane, indicating increased anterior-posterior movement between the pelvis and thigh [
33, 34], possibly as a compensatory mechanism to accommodate altered lower limb alignment and provide stability during weight-bearing activities. However, in the transverse and frontal planes, DP values are significantly reduced, indicating limited lateral and rotational stability, which may contribute to the observed knee valgus alignment [
24].
During the swing phase, female athletes with DKV exhibit higher DP values in the sagittal and transverse planes in pelvic-thigh coordination, indicating altered hip flexion and extension dynamics that may influence overall gait patterns and efficiency. DP values are significantly lower in the frontal plane, which can contribute to DKV by allowing inward knee collapse during the swing phase [
33, 34].
Significant differences were observed in MARP values in the frontal and transverse planes between the shank and thigh during both phases of gait. Greater MARP values in the coronal plane during the stance phase indicate less coordinated movement between the thigh and shank, which potentially contributes to instability during weight-bearing activities [
33, 34] and DKV [
31]. In the swing phase, lower MARP values in the transverse plane indicate reduced coordination that may affect proper foot positioning and stability during subsequent stance, further exacerbating the knee valgus alignment [
33, 34]. Regarding thigh-shank coordination, the current study examined thigh-shank coordination in female athletes with DKV during both phases of walking gait. During the stance phase, DKV athletes showed a significant reduction in mean DP values in the transverse and frontal planes, indicating decreased coordinated movement between the thigh and shank in lateral and rotational planes [
35, 36]. This reduced coordination may be linked to limited lateral stability and rotational control of the lower limb [
24], potentially contributing to the observed DKV alignment during stance. In addition, during the swing phase, DKV athletes exhibited significantly higher DP values in the frontal plane and lower DP values in the transverse planes, which may demonstrate a compensatory mechanism to adapt to altered lower limb mechanics during this phase [
31, 32]. However, the reduced transverse plane coordination during swing may influence foot positioning and stability during the subsequent stance phase, potentially impacting the knee valgus alignment [
35, 36].
Moreover, the study identified notable differences in thigh-shank coordination during the swing phase. DKV athletes displayed significantly higher MARP values in the sagittal and transverse planes, indicating altered hip and knee movement patterns that may affect overall gait efficiency and potentially contribute to the DKV observed in these athletes. The higher MARP values in the transverse plane may indicate excessive pelvic rotation relative to the thigh during the swing, affecting foot positioning for the subsequent stance phase [
35, 36]. Conversely, lower MARP values in the frontal plane during swing indicate reduced coordination between the thigh and shank, possibly contributing to inward knee collapse during the swing phase [
24], further accentuating the DKV in female athletes. These changes may explain the enhanced risk of sustaining more ACL damage in athletes with developmental knee valgus [
1,
37].
The present study investigated shank-foot coordination in women athletes with DKV during gait. DKV athletes showed significantly higher DP values in the frontal, sagittal, and transverse planes in the stance phase, indicating increased movement variability and deviations between the shank and foot segments [
38]. This may be associated with altered lower limb alignment, potentially contributing to the DKV observed during stance [
38, 39]. Conversely, during the swing phase, DKV athletes exhibited significantly lower DP values in all three planes, indicating reduced movement variability and deviations, potentially as a compensatory mechanism to maintain stability and control throughout the gait cycle [
38, 39].
Regarding to MARP values in shank-foot coordination, DKV athletes displayed significantly higher coordination and synchronization between these segments in all planes during the stance phase. This heightened coordination during stance may serve to stabilize the lower limb and counter the impact of DKV during this phase [
38, 39]. However, during the swing phase, DKV athletes exhibited significantly lower MARP values in all three planes, indicating reduced coordination and synchronization between the shank and foot segments. This decreased coordination during swing may influence foot positioning and overall lower limb stability during the transition from swing to stance and could be a participating element to the observed DKV during walking [
24,
31, 32].
In summary, the current study provides valuable insights into the intersegmental coordination patterns of female athletes with DKV during walking. The results highlight significant differences in thigh-shank, shank-foot, and pelvic-thigh coordination in cardinal planes between athletes with and without DKV. These results can be useful for developing targeted interventions and training strategies to address the altered coordination patterns and reduce injury risk in women athletes with DKV. According to the results of this research, corrective protocols or preventive measures for athletes with DKV should be designed to address the coordination patterns of all the lower extremity joints. Establishing stability and coordination between the proximal and distal segments is equally crucial in addressing the biomechanical changes at the knee to prevent injuries in DKV-afflicted athletes. However, further study is needed to explore the causal connection between the observed coordination patterns and the development of DKV, as well as to validate the effectiveness of potential intervention strategies.
The present study’s results have limitations that emphasize the necessity for further research to authenticate the reported results. For instance, the study did not focus on participants from specific sports disciplines, which makes it difficult to generalize the findings to all athletes since different sports have varying requirements. In addition, the discrepancies in landing mechanics between the two genders indicate that the results may not apply to male athletes. Furthermore, the cross-sectional design of the study leaves the real impact of cognitive load on knee injuries yet to be determined by future studies. Moreover, participants were asked to walk barefoot to minimize the potential influence of shoe type on the study results. However, it is essential to recognize that in real sports scenarios, athletes engage in more challenging movements, such as jumping and landing, and the type of footwear can significantly affect landing mechanics. Therefore, it is critical to consider these factors for the application of the study’s findings in practical sports settings.
Conclusion
In conclusion, the current study demonstrates that female athletes with DKV exhibit distinct intersegmental coordination patterns in the pelvis- thigh, shank-foot, and thigh-shank during walking. Understanding these biomechanical alterations can guide the development of targeted interventions aimed at improving lower limb stability, reducing injury risk, and optimizing athletic performance in female athletes with DKV. Further research is needed to explore the causal connection between these coordination patterns and DKV development, as well as to evaluate the effectiveness of risk reduction intervention strategies.
Ethical Considerations
Compliance with ethical guidelines
This study was approved by the Biomedical Research Ethics Board of Allameh Tabataba’i University (Code: IR.ATU.REC.1401.048).
Funding
This manuscript is drafted from the master's thesis of Mina Zamankhanpour, approved by the Department of Corrective Exercise & Sports Injury, Faculty of Physical Education and Sport Sciences,Allameh Tabataba’i University, Tehran, Iran.
Authors' contributions
Study design and data collection: Mina Zamankhanpour, Rahman Sheikhhoseini, Hashem Piri, Shakiba Asadi_Melerdi, and Sajjad Abdollahi; Writing: Mina Zamankhanpour, Rahman Sheikhhoseini and Timothy E. Hewett, Final approval: All authors.
Conflict of interest
The authors declared no conflict of interest.
References
- Hewett TE, Myer GD, Ford KR, Heidt RS Jr, Colosimo AJ, McLean SG, et al. Biomechanical measures of neuromuscular control and valgus loading of the knee predict anterior cruciate ligament injury risk in female athletes: A prospective study. The American Journal of Sports Medicine. 2005; 33(4):492-501. [DOI:10.1177/0363546504269591] [PMID]
- Myer GD, Ford KR, Di Stasi SL, Foss KD, Micheli LJ, Hewett TE. High knee abduction moments are common risk factors for patellofemoral pain (PFP) and anterior cruciate ligament (ACL) injury in girls: Is PFP itself a predictor for subsequent ACL injury? British Journal of Sports Medicine. 2015; 49(2):118-22. [DOI:10.1136/bjsports-2013-092536] [PMID]
- Schmitz RJ, Shultz SJ, Nguyen AD. Dynamic valgus alignment and functional strength in males and females during maturation. Journal of Athletic Training. 2009; 44(1):26-32. [DOI:10.4085/1062-6050-44.1.26] [PMID]
- Xu D, Lu J, Baker JS, Fekete G, Gu Y. Temporal kinematic and kinetics differences throughout different landing ways following volleyball spike shots. Proceedings of the Institution of Mechanical Engineers, Part P: Journal of Sports Engineering and Technology. 2022; 236(3):200-8. [DOI:10.1177/17543371211009485]
- Hewett TE, Myer GD, Ford KR. Anterior cruciate ligament injuries in female athletes: Part 1, mechanisms and risk factors. The American Journal of Sports Medicine. 2006; 34(2):299-311. [DOI:10.1177/0363546505284183] [PMID]
- Holden S, Boreham C, Delahunt E. Sex differences in landing biomechanics and postural stability during adolescence: A systematic review with meta-analyses. Sports Medicine. 2016; 46(2):241-53. [DOI:10.1007/s40279-015-0416-6] [PMID]
- Dugan SA. Sports-related knee injuries in female athletes: What gives? American Journal of Physical Medicine & Rehabilitation. 2005; 84(2):122-30. [DOI:10.1097/01.PHM.0000154183.40640.93] [PMID]
- Russell KA, Palmieri RM, Zinder SM, Ingersoll CD. Sex differences in valgus knee angle during a single-leg drop jump. Journal of Athletic Training. 2006; 41(2):166-71. [PMID]
- Wilczyński B, Zorena K, Ślęzak D. Dynamic knee valgus in single-leg movement tasks. Potentially modifiable factors and exercise training options. A literature review. International Journal of Environmental Research and Public Health. 2020; 17(21):8208. [DOI:10.3390/ijerph17218208] [PMID]
- Carson DW, Ford KR. Sex differences in knee abduction during landing: A systematic review. Sports Health. 2011; 3(4):373-82. [DOI:10.1177/1941738111410180] [PMID]
- Merkel DL. Youth sport: Positive and negative impact on young athletes. Open Access Journal of Sports Medicine. 2013; 4:151-60. [DOI:10.2147/OAJSM.S33556] [PMID]
- Yang NH, Nayeb‐Hashemi H, Canavan PK, Vaziri A. Effect of frontal plane tibiofemoral angle on the stress and strain at the knee cartilage during the stance phase of gait. Journal of Orthopaedic Research. 2010; 28(12):1539-47. [DOI:10.1002/jor.21174] [PMID]
- Hoch MC, Weinhandl JT. Effect of valgus knee alignment on gait biomechanics in healthy women. Journal of Electromyography and Kinesiology. 2017; 35:17-23. [DOI:10.1016/j.jelekin.2017.05.003] [PMID]
- Barrios JA, Heitkamp CA, Smith BP, Sturgeon MM, Suckow DW, Sutton CR. Three-dimensional hip and knee kinematics during walking, running, and single-limb drop landing in females with and without genu valgum. Clinical Biomechanics. 2016; 31:7-11. [DOI:10.1016/j.clinbiomech.2015.10.008] [PMID]
- Winter DA. Foot trajectory in human gait: A precise and multifactorial motor control task. Physical Therapy. 1992; 72(1):45-53. [DOI:10.1093/ptj/72.1.45] [PMID]
- Harbourne RT, Stergiou N. Movement variability and the use of nonlinear tools: Principles to guide physical therapist practice. Physical Therapy. 2009; 89(3):267-82. [DOI:10.2522/ptj.20080130] [PMID]
- Hausdorff JM, Schweiger A, Herman T, Yogev-Seligmann G, Giladi N. Dual-task decrements in gait: Contributing factors among healthy older adults. The Journals of Gerontology Series A: Biological Sciences and Medical Sciences. 2008; 63(12):1335-43. [DOI:10.1093/gerona/63.12.1335] [PMID]
- Liu-Ambrose T, Katarynych LA, Ashe MC, Nagamatsu LS, Hsu CL. Dual-task gait performance among community-dwelling senior women: The role of balance confidence and executive functions. Journals of Gerontology Series A: Biomedical Sciences and Medical Sciences. 2009; 64(9):975-82. [DOI:10.1093/gerona/glp063] [PMID]
- Kimura A, Yokozawa T, Ozaki H. Clarifying the biomechanical concept of coordination through comparison with coordination in motor control. Frontiers in Sports and Active Living. 2021; 3:753062. [DOI:10.3389/fspor.2021.753062][PMID]
- Stergiou N. Innovative analyses of human movement. Champaign: Human Kinetics Champaign; 2004. [Link]
- Hafer JF, Boyer KA. Variability of segment coordination using a vector coding technique: Reliability analysis for treadmill walking and running. Gait & Posture. 2017; 51:222-7. [DOI:10.1016/j.gaitpost.2016.11.004] [PMID]
- Preatoni E, Hamill J, Harrison AJ, Hayes K, Van Emmerik RE, Wilson C, et al. Movement variability and skills monitoring in sports. Sports Biomechanics. 2013; 12(2):69-92. [DOI:10.1080/14763141.2012.738700] [PMID]
- Hamill J, Palmer C, Van Emmerik RE. Coordinative variability and overuse injury. Sports Medicine, Arthroscopy, Rehabilitation, Therapy & Technology. 2012; 4(1):45. [DOI:10.1186/1758-2555-4-45] [PMID]
- Larwa J, Stoy C, Chafetz RS, Boniello M, Franklin C. Stiff landings, core stability, and dynamic knee valgus: A systematic review on documented anterior cruciate ligament ruptures in male and female athletes. International Journal of Environmental Research and Public Health. 2021; 18(7):3826.[DOI:10.3390/ijerph18073826][PMID]
- Desailly E, Daniel Y, Sardain P, Lacouture P. Foot contact event detection using kinematic data in cerebral palsy children and normal adults gait. Gait & Posture. 2009; 29(1):76-80. [DOI:10.1016/j.gaitpost.2008.06.009] [PMID]
- Chiu SL, Chou LS. Effect of walking speed on inter-joint coordination differs between young and elderly adults. Journal of Biomechanics. 2012; 45(2):275-80. [DOI:10.1016/j.jbiomech.2011.10.028] [PMID]
- Seay JF, Van Emmerik RE, Hamill J. Low back pain status affects pelvis-trunk coordination and variability during walking and running. Clinical Biomechanics. 2011; 26(6):572-8. [DOI:10.1016/j.clinbiomech.2010.11.012] [PMID]
- Hamill J, van Emmerik RE, Heiderscheit BC, Li L. A dynamical systems approach to lower extremity running injuries. Clinical Biomechanics. 1999; 14(5):297-308. [DOI:10.1016/S0268-0033(98)90092-4]
- Meyns P, Van Gestel L, Bruijn SM, Desloovere K, Swinnen SP, Duysens J. Is interlimb coordination during walking preserved in children with cerebral palsy? Research in Developmental Disabilities. 2012; 33(5):1418-28. [DOI:10.1016/j.ridd.2012.03.020] [PMID]
- Stergiou N, Jensen JL, Bates BT, Scholten SD, Tzetzis G. A dynamical systems investigation of lower extremity coordination during running over obstacles. Clinical Biomechanics. 2001; 16(3):213-21. [DOI:10.1016/S0268-0033(00)00090-5]
- Dadfar M, Sheikhhoseini R, Jafarian M, Esmaeili A. Lower extremity kinematic coupling during single and double leg landing and gait in female junior athletes with dynamic knee valgus. BMC Sports Science, Medicine and Rehabilitation. 2021; 13(1):152. [DOI:10.1186/s13102-021-00385-y] [PMID]
- Dadfar M, Soltani M, Novinzad MB, Raahemifar K. Lower extremity energy absorption strategies at different phases during single and double-leg landings with knee valgus in pubertal female athletes. Scientific Reports. 2021; 11(1):17516. [DOI:10.1038/s41598-021-96919-y] [PMID]
- Ebrahimi S, Kamali F, Razeghi M, Haghpanah SA. Correlation between Trunk-Pelvis Inter-Segmental Coordination Parameters during Walking and Disability Level in Chronic Low Back Pain Patients. Journal of Biomedical Physics & Engineering. 2018; 8(2):193-202. [DOI:10.31661/jbpe.v8i2.602]
- Blandford L, Cushion E, Mahaffey R. Segmental and intersegmental coordination characteristics of a cognitive movement control test: Quantifying loss of movement choices. Biomechanics. 2022; 2(2):213-34. [DOI:10.3390/biomechanics2020018]
- Dewolf AH, Meurisse GM, Schepens B, Willems PA. Effect of walking speed on the intersegmental coordination of lower-limb segments in elderly adults. Gait & Posture. 2019; 70:156-61. [DOI:10.1016/j.gaitpost.2019.03.001] [PMID]
- Ebrahimi S, Kamali F, Razeghi M, Haghpanah SA. Comparison of the trunk-pelvis and lower extremities sagittal plane inter-segmental coordination and variability during walking in persons with and without chronic low back pain. Human Movement Science. 2017; 52:55-66. [DOI:10.1016/j.humov.2017.01.004] [PMID]
- Krosshaug T, Nakamae A, Boden BP, Engebretsen L, Smith G, Slauterbeck JR, et al. Mechanisms of anterior cruciate ligament injury in basketball: video analysis of 39 cases. The American Journal of Sports Medicine. 2007; 35(3):359-67. [DOI:10.1177/0363546506293899] [PMID]
- Mazurek CM, Pearsall DJ, Renaud PJ, Robbins SM. Differences in inter-segment coordination between high- and low-calibre ice hockey players during forward skating. Sports Biomechanics. 2020; 22:1303-18. [DOI:10.1080/14763141.2020.1797151]
- Donaldson BJ, Bezodis NE, Bayne H. Inter- and intra-limb coordination during initial sprint acceleration. Biology Open. 2022; 11(10):bio059501. [DOI:10.1242/bio.059501] [PMID]