Introduction
The knee joint, situated between the longest lever arms (the thigh and shank) and supported by the body’s strongest muscles, is among the joints subjected to substantial forces, making alterations in its stability probable [
1].
Abnormal neuromuscular performance of the lower limbs can increase the degree of knee valgus and subsequently the risk of anterior cruciate ligament (ACL) injury [
2, 3]. In other words, neuromuscular dysfunctions are a major contributor to non-contact ACL injuries in athletes, increasing the load on the lower limb joints during physical activities, thereby increasing the risk of ACL injury [
4]. Palmer-Smith et al., in a study on the relationship between feed-forward activity of the muscles around the knee and peak knee valgus angle, maintained that the peak knee valgus angle in women is associated with increased activity in the external hip rotator and hamstring muscles, and reducing the knee valgus angle is associated with intensified feedforward activity of the external hip rotator muscles during landing, indicating that restoring the balance between internal and external thigh muscles in women can help reduce knee valgus, hence potentially reducing the risk of ACL injury [
5]. Hewett et al. (2010), in a video analysis study of non-contact ACL injuries in athletes, identified four neuromuscular deficits as foundational mechanisms, including ligament dominance (valgus collapse), quadriceps dominance, foot dominance, and trunk dominance [
6]. In the current research, among the common mechanisms of non-contact ACL injuries, dynamic knee valgus has been selected due to its strong association with non-contact ACL injuries [
2]. Dynamic knee valgus during landing from jumps or squats is a combination of femoral adduction and internal rotation with tibial abduction and external rotation [
7]. Essentially, knee valgus results from the coordination of movements between the femur and tibia, affecting proximal and distal knee joints, including the trunk, thigh, and ankle [
7]. Studies on cadavers have shown that the knee valgus pattern, especially in combination with substantial internal rotation, increases the strain on the ACL [
8]. Accordingly, dynamic knee valgus motion is often considered a key risk factor in acute knee injuries. Reports suggest that increased motion in dynamic knee valgus during dynamic activities (increased medial collapse during functional activities, such as landing, running, etc.) may be associated with increased lower limb injuries, leading to excessive stress on the knee joint or hip joint [
9]. Evidence suggests that the majority of basketball injuries (58% to 66%) occur in the lower extremities [
10]. These injuries negatively impact players’ performance upon returning to the game, potentially constituting more than one-fourth of lost games in professional athletes due to injuries [
11]. Interest in the type and mechanism of basketball-related injuries is continually increasing [
12]. Jumping and landing are common actions in basketball, and various injuries may occur during their execution. Previous studies supporting the hypothesis that players’ positions in basketball teams affect their injury rates have led to disagreements among researchers regarding which player positions are more susceptible to injuries. Yde et al. (2006), in their studies on basketball players, concluded that forwards and subsequently centers experienced higher injury rates compared to other positions [
13].
Considering that the duties, physiology, type, intensity, and level of physical activity, anthropometric characteristics, muscle strength, and flexibility of players vary across different positions [
14], there has been limited research on the impact of player positions on the occurrence and nature of their injuries. It is expected that injury patterns and rates differ among players in various positions. There are conflicting findings in this area, and a consensus among researchers on which position is most prone to injuries has not been reached. Limited information is available regarding injury occurrence in different positions due to the physiological and anthropometric conditions of players, as well as the specific roles and responsibilities associated with each position. On the other hand, sufficient information in this field is lacking, as researchers have demonstrated that the incidence of injuries and influencing factors varies across different countries and regions worldwide [
15, 16]. Given the differences in playing styles in each country, conducting such research seems necessary. Therefore, the current study compares the lower limb joint angles in basketball players with dynamic knee valgus deficiency across different positions.
Materials and Methods
The research population for this study consisted of semi-professional male basketball players with dynamic knee valgus flaws, aged between 16 and 26, from Kermanshah Province, Iran. In this study, 27 basketball players with dynamic knee valgus flaws were purposefully selected and categorized into three groups as follows: Guards, forwards, and centers. The selection process was non-randomized but purposeful, aiming to represent various playing positions within the study population. Before the commencement of the research, the participants completed a questionnaire covering medical and sports information, along with a consent form. The Code of Ethics has also been received from the University of Guilan. During the explanatory session held before the study at the testing site, sufficient information about the research was provided to the participants, assuring them that the present study posed no health risks to them. The participants were required to engage in basketball training sessions at least three times a week. However, those who had experienced injury or surgery, significant cardiovascular, respiratory, or neurological disorders in the past six months, or had structural valgus or varus patterns were excluded from the study.
Anthropometric characteristics, including the participant’s height and weight, were measured using a standing digital height gauge (Inbody BSM 170, Japan) and a smart scale (Mi-Smart-Scale 2, China). Additionally, shoulder width, hip width, leg length, medial and lateral knee condyle width, external and internal malleoli distance, and Q angle were measured using a measuring tape, calipers (Mitutoyo, Japan), and a goniometer. The plug-in gait marker method was also utilized for the lower limbs, involving 20 markers per individual [
17, 18]. Reflex markers were placed on the lateral malleolus, posterior heel, between the first and second metatarsal joints, lateral side of the shank, lateral condyle of the knee, patellar center, lateral side of the thigh, greater trochanter of the hip, anterior superior iliac spine, and posterior superior iliac spine on both sides. For initial screening, we employed the Tack and Cham test to diagnose knee dynamic patterns. To perform the tuck jump, the athlete stands with feet shoulder-width apart, initiates a vertical jump with legs fully extended, and elevates the knees as high as possible. At the peak of the jump, thighs should be parallel to the ground. Upon landing, the athlete prepares for the next tuck jump. The test was executed for 10 s [
19]. Qualified participants were required to clear the shin of the trailing leg over a horizontal line during the jumping mechanography test [
20]. After the selection of eligible players, each one prepared at the sports facility’s dedicated basketball court at 5:00 PM with prior coordination. The angles were recorded using two filming cameras, adjusted based on the participant’s height, and oriented parallel to the frontal and sagittal planes [
21]. A 10-min general warm-up was initially implemented for the players. Subsequently, participants were instructed to stand in the designated area and perform three countermovement jumps [
22]. The average of the three jumps was captured by the cameras. After the test, the Kinovea software was employed to measure joint angles of the hip, knee, and ankle.
Angles of hip flexion and extension in the sagittal plane were determined by identifying markers placed on the anterior superior iliac spine, greater trochanter of the hip, and mid-thigh (
Figure 1).
Knee flexion and extension angles on the sagittal plane were obtained using markers on the mid-thigh, lateral condyle of the knee, and mid-tibia (
Figure 2).
Knee valgus and varus patterns in the frontal plane were assessed through markers on the anterior superior iliac spine, middle of the patella, and second metatarsal (
Figure 3).
Ankle dorsiflexion and plantarflexion angles were derived by identifying markers on the mid-tibia, lateral malleolus, and second metatarsal using the Kinovea software (
Figure 4) [
23].
To examine the moment of initial contact and the deepest flexion of the hip, knee, and ankle joints during landing, the first frame from the start of the movement was selected. Then, an angle was drawn using three points for the hip joint (anterior superior iliac spine, greater trochanter of the hip, and mid-thigh), knee joint (mid-thigh, lateral condyle of the knee, and mid-tibia), and ankle joint (mid-tibia, lateral malleolus, and second metatarsal). Subsequently, the last frame during landing was chosen, and again, an angle was drawn according to the figure. Finally, the joint angle at the initial contact (first frame of the push-off phase) was subtracted from the joint angle at landing (last frame of the landing phase at initial contact and maximum flexion) to obtain the initial contact and deepest flexion angles of the hip, knee, and ankle joints.
Similarly, to examine the moment of initial contact and the deepest flexion of the knee joint in the frontal plane during landing, the first frame from the start of the movement was chosen. Then, an angle was drawn using three points for the knee joint (anterior superior iliac spine, middle of the patella, and second metatarsal). Following the previous steps, the last frame during landing was selected, and again, an angle was drawn according to the figure. Finally, the joint angle at the initial contact (first frame of the push-off phase) was subtracted from the joint angle at landing (last frame of the landing phase at initial contact and maximum flexion) to obtain the initial contact and deepest flexion angles of the knee joint in the frontal plane.
Statistical method
To analyze the raw data obtained from the research, both descriptive and inferential statistics were employed. Descriptive statistics, including Mean±SD, were used to describe the demographic characteristics of the participants. The normality of data distribution was assessed using the Shapiro-Wilk test. In the case of normal distribution, a one-way analysis of variance test was utilized for each movement task. Finally, the raw data from the study were summarized in Excel and analyzed using the SPSS software, version 23 (IBM Corp, Redmond, WA, USA). The significance level in this study is set at 95%, with an α level of P≤0.05.
Results
The Mean±SD of the participant’s demographic characteristics, including age, weight, height, body mass index, shoulder width, hip width, anterior superior iliac spine distance, knee condyle width, ankle width, lower limb length, and Q angle, are listed in
Table 1.
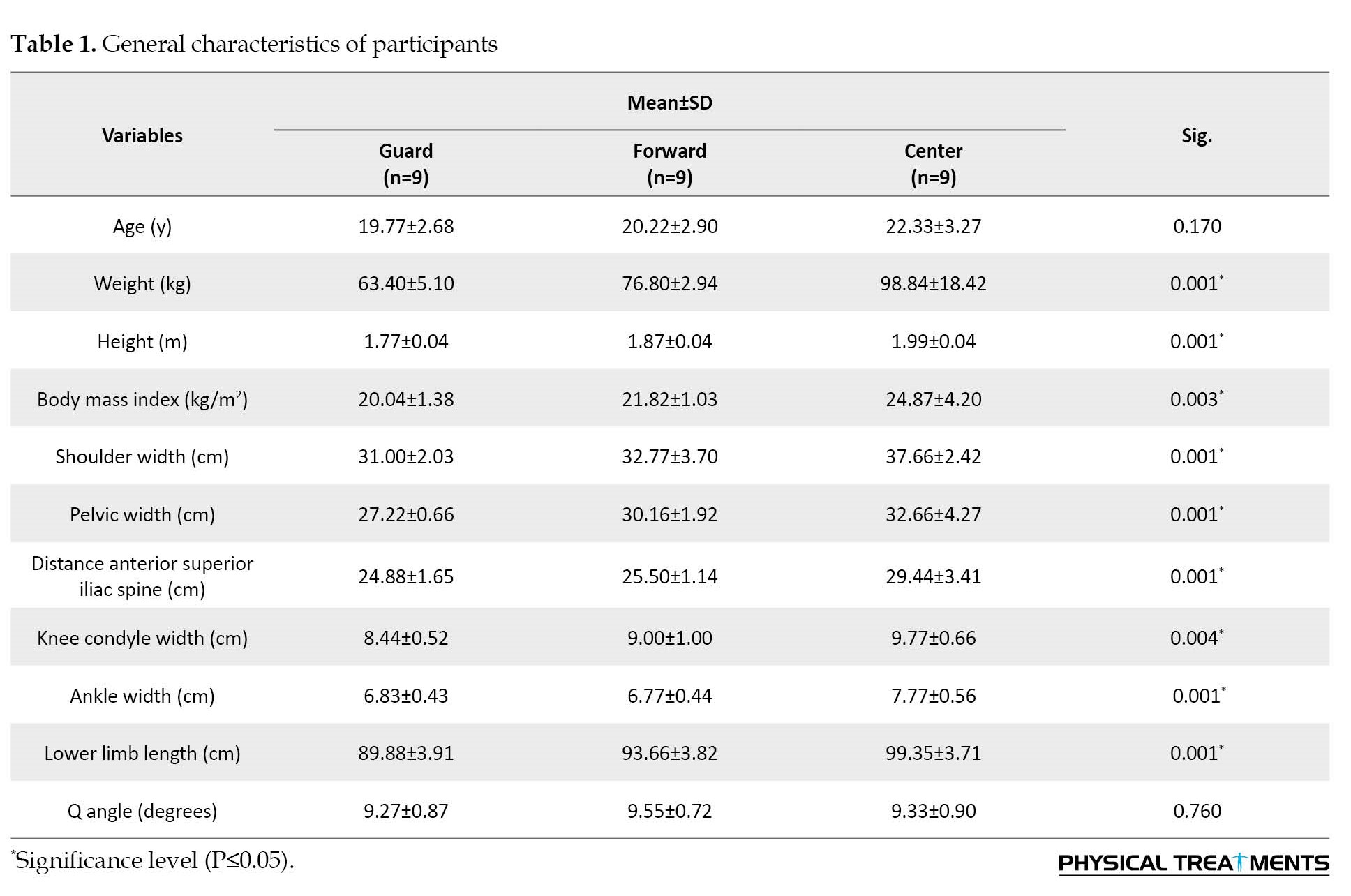
In this section, the general characteristics of the participants are presented separately in
Table 1. The results of the analysis of variance test indicate that in the personal characteristics, variables of age and Q angle are homogeneously distributed across the three groups, while other variables are not homogeneously distributed across the three groups.
Descriptive statistics (Mean±SD and confidence interval) for the research variables, separated by each group, are presented in
Table 2 for the pre-test.
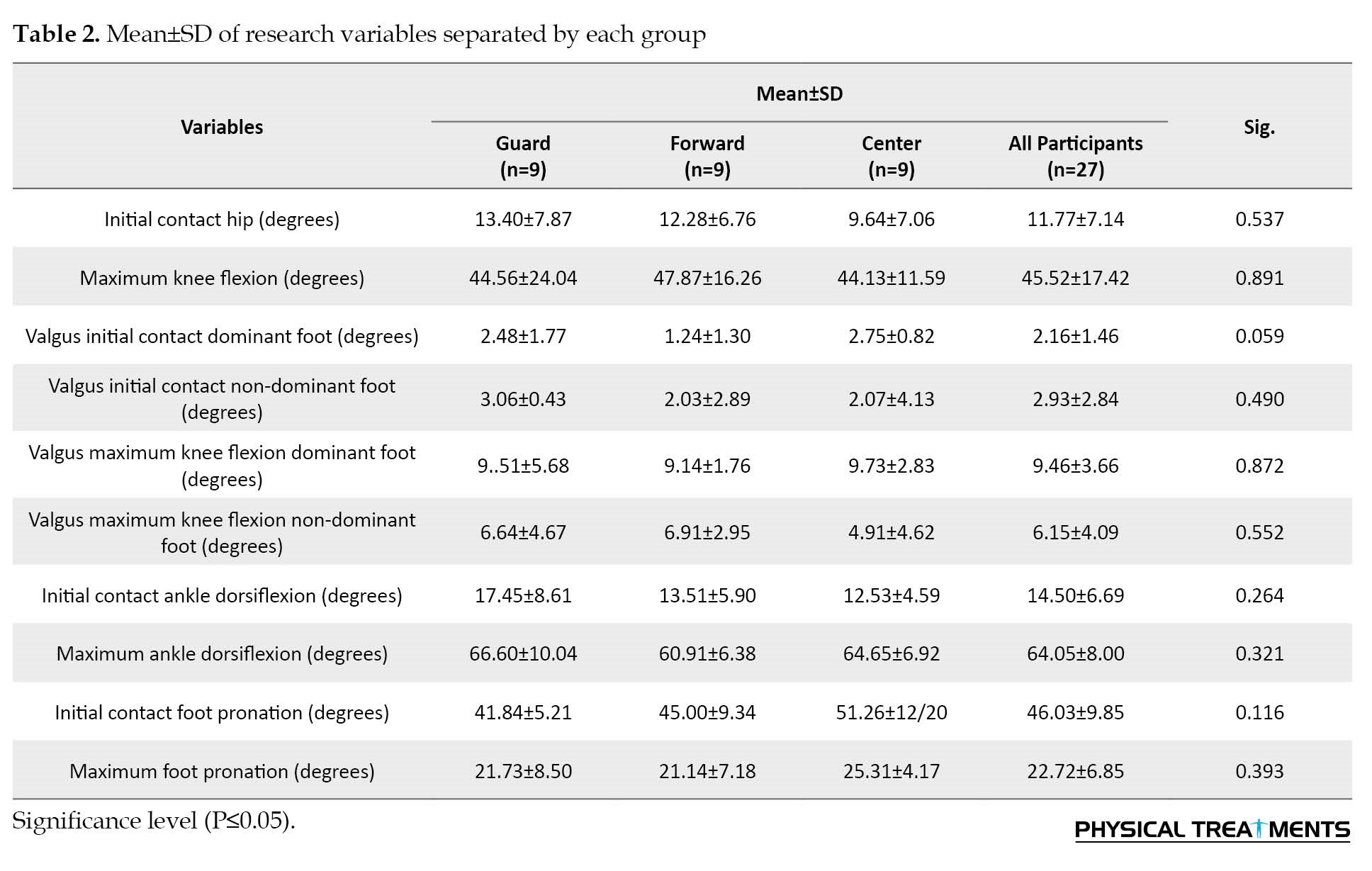
Descriptive statistics (Mean±SD) for the research variables, separated by each group, are presented in
Table 2. The analysis of variance test results for inter-group comparison of the means of research variables indicated no significant differences among the variables in the three groups (
Table 3).
Discussion
The current study compared the lower limb joint angles in basketball players with knee dynamic valgus patterns in different positions. The results showed no significant difference in the angles of the lower limb joints among the three following groups: Guard, forward, and center. In this study, no significant differences were found in the joint angles of the thigh, knee, and ankle among different basketball positions. Due to the high prevalence of non-contact injuries in basketball, particularly in the lower extremities, this study investigated whether player positions could serve as a risk factor for these injuries. According to the results obtained, no significant differences were found among players of different positions. However, other variables, such as fatigue and positional responsibilities during gameplay may contribute to differences in injury rates among players in various positions. While limited quantitative research has been conducted on different positions in this field, a few studies provide insights into this matter.
In the present study, basketball players from different positions exhibited physiological and anatomical differences. In a study by Sallet et al. (2005), it was noted that centers were taller and heavier than forwards, and forwards were taller and heavier than guards [
24]. Rismayadi et al. (2023) conducted a study titled “differences in physical conditions for each playing position in basketball athletes.” The results of the study showed a significant difference in the overall physical condition of each playing position. The point guard, shooting guard, and small forward positions do not differ in physical condition, while only power forwards and centers tend to have different physical conditions from point guards. Therefore, the positions can be grouped into the three following parts: The small forward position has no difference in physical condition from other positions, the point guard and shooting guard positions have different physical conditions than the power forward and center, and the power forward and center have different physical conditions than the point guard and shooting guard [
25]. Delextrat et al. (2009) conducted a study titled “strength, power, speed, and agility of women basketball players according to playing position.” The study was to investigate the effect of playing position on the strength, power, speed, and agility performances of women basketball players. Thirty subjects playing at the national level participated in this study. They were divided into 3 groups according to playing position: Guards (positions 1 and 2), forwards (positions 3 and 4), and centers (position 5). Each subject performed 8 tests presented in random order: The 30-s Wingate anaerobic test, isokinetic testing of the knee extensors, 2 types of jump tests, a 20-m sprint, the agility t-test, a suicide run, and a basketball chest pass. Statistical differences between playing positions were assessed using a one-way analysis of variance and Scheffe post hoc analyses. Results showed that guards performed significantly better than centers for the relative peak and mean power achieved during the Wingate anaerobic test (+13% and +16.9%, respectively), relative peak torque of knee extensors (+19.5%), single-leg jump (+21.8), suicide run (+7.5%), and agility t-test (+6.4%, P<0.05). In addition, guards achieved significantly better performances than forwards in the suicide run test (+7.1%) and forwards were characterized by greater peak torque of the knee extensors compared to centers (+22.1%). These results indicate that specific fitness training must be undertaken according to playing position. The ability to perform the suicide run, the single-leg jump, and the different movements involved in the agility t-test must be developed in guards. In contrast, speed over short distances and strength development of the lower body and upper body should be performed by all playing positions [
26]. Based on the conducted research and the existence of differences in variables such as physical readiness, strength, speed, and agility among players in different positions, there was a possibility that differences might exist in the kinematic angles of players during landing from jumps. However, the results of this study did not demonstrate any differences in this parameter. In this regard, Belali Shamsara and colleagues (2013) [
27] conducted a study titled “the effect of football player positions in the Iran pro league on the incidence of sports injuries” for this purpose, video footage of 125 matches out of 240 in the 86-85 season was analyzed by one of the researchers. Information related to the players’ positions and their injuries was recorded. A total of 306 injuries occurred in these 125 matches, and midfielders (24.51%) significantly suffered more injuries than players in other positions. Additionally, forwards (18.88%) and midfielders (17.72%) were more prone to injuries during attacks, while defenders (15.86%) and goalkeepers (9.91%) experienced more injuries during defense. Most injuries (61.8%) resulted from player-to-player collisions, and the lower extremities (61.8%) were more exposed to injuries than other body parts. The results of this study indicated that the incidence of injuries, the mechanism of injury occurrence, and the exposed area to injuries differ among various playing positions [
27]. In another study, Balali, Shamsara, et al. (2013) conducted research titled “investigating the effect of player positions in the Iranian men’s basketball league on the incidence of sports injuries.” Detailed information about the results of this research has not been provided. To achieve this, 100 video clips from competitions in the 86-87 season were obtained and analyzed by one of the researchers. Information regarding players’ positions and their injuries was recorded. In total, 55 injuries occurred in these 100 matches, and forwards (40%) significantly suffered more injuries than other positions. The majority of injuries resulted from direct collisions (72.72%). There was a significant difference in the incidence of injuries between visiting and home teams. The results of this study demonstrated that the incidence of injuries, their mechanism, and the exposed area to injuries vary across different basketball positions [
28]. In the aforementioned study, the majority of reported injuries across different basketball positions were collision-related (72.72%). This factor contributed to the observed differences among various player positions. In contrast, in the current research, the lack of differences in the angles of lower limb joints across different basketball positions was reported as a contributing factor to non-collision injuries. In a study conducted by Moreira et al. (2003) on the Brazilian national team, it was observed that only two cases of injuries were related to the head region, with one case involving a guard player and the other a center player. Among the reported injuries, 9.40% were attributed to center players, and 7.22% were associated with guard players [
29]. Krosshaug et al. (2005), through an analysis of NBA men’s and women’s basketball game footage, reported injuries occurring during offense in 29 cases, defense in five cases, and rebound situations in two cases [
30]. In another study, Perše et al. (2006) found a higher incidence of injuries among forward and center players compared to other positions in basketball [
31]. Erčulj et al. (2006), in a study on basketball players, reported a higher incidence of injuries among forward players [
31]. In the research conducted, the analysis of results was based on game videos, where the mechanism, type, and location of injuries were reported. However, in the current study, the angles of lower limb joints during landing among players in different positions have been examined and showed no significant differences. Therefore, it can be concluded that different player positions may not act as a significant risk factor for non-contact ACL injuries.
Conclusion
In summary, the overall results of this research indicate no differences in the angles of lower limb joints among basketball players in the three positions of guard, forward, and center. The findings suggest that players in different positions in the sport of basketball did not exhibit variations in the kinematic angles of their lower limb joints. This factor may not significantly contribute as a risk factor for non-contact ACL injuries. However, basketball players may experience varying levels of injury due to different positional responsibilities and functions during the game or fatigue towards the end of the game.
Ethical Considerations
Compliance with ethical guidelines
This study was approved by the Ethics Committee of the University of Guilan (Code: IR.GUILAN.REC.1402.083).
Funding
The paper was extracted from the master's thesis of Rashin Asadpour, approved by Department of Corrective Exercise and Sport Injuries, Faculty of Physical Education and Sport Science, University of Guilan.
Authors' contributions
All authors contributed equally to preparing this article.
Conflict of interest
The authors declared no conflict of interest.
Acknowledgments
The authors express their gratitude to all people who participated in the present research.
References
- Ghasemi F, Amiri A, Maarufi N, Jamshidi AA, Jalaei S. [Reliability of onset muscle activity of the knee joint on the exposure of unexpected rotary turbulence (Persian)]. Journal of Modern Rehabilitation. 2016; 9(S1):1-11. [Link]
- Ford KR, Myer GD, Hewett TE. Valgus knee motion during landing in high school female and male basketball players. Medicine and Science in Sports and Exercise. 2003; 35(10):1745-50. [DOI:10.1249/01.MSS.0000089346.85744.D9] [PMID]
- Myer GD, Ford KR, Brent JL, Hewett TE. Differential neuromuscular training effects on ACL injury risk factors in"high-risk" versus "low-risk" athletes. BMC Musculoskeletal Disorders. 2007; 8:39. [DOI:10.1186/1471-2474-8-39] [PMID] [PMCID]
- Hewett TE, Myer GD, Ford KR, Heidt RS Jr, Colosimo AJ, McLean SG, et al. Biomechanical measures of neuromuscular control and valgus loading of the knee predict anterior cruciate ligament injury risk in female athletes: A prospective study. The American Journal of Sports Medicine. 2005; 33(4):492-501. [DOI:10.1177/0363546504269591] [PMID]
- Palmieri-Smith RM, McLean SG, Ashton-Miller JA, Wojtys EM. Association of quadriceps and hamstrings cocontraction patterns with knee joint loading. Journal of Athletic Training. 2009; 44(3):256-63. [DOI:10.4085/1062-6050-44.3.256] [PMID] [PMCID]
- Hewett TE, Ford KR, Hoogenboom BJ, Myer GD. Understanding and preventing acl injuries: Current biomechanical and epidemiologic considerations-Update 2010. North American Journal of Sports Physical Therapy. 2010; 5(4):234-51. [PMID] [PMCID]
- Barrios JA, Heitkamp CA, Smith BP, Sturgeon MM, Suckow DW, Sutton CR. Three-dimensional hip and knee kinematics during walking, running, and single-limb drop landing in females with and without genu valgum. Clinical Biomechanics. 2016; 31:7-11. [DOI:10.1016/j.clinbiomech.2015.10.008] [PMID]
- Lloyd DG. Rationale for training programs to reduce anterior cruciate ligament injuries in Australian football. The Journal of Orthopaedic and Sports Physical Therapy. 2001; 31(11):645-54. [DOI:10.2519/jospt.2001.31.11.645] [PMID]
- Nakagawa TH, Petersen RS. Relationship of hip and ankle range of motion, trunk muscle endurance with knee valgus and dynamic balance in males. Physical Therapy in Sport. 2018; 34:174-9. [DOI:10.1016/j.ptsp.2018.10.006] [PMID]
- Edery D, Mollick E. Changing the game: How video games are transforming the future of business. Saddle River: Pearson Education; 2008. [Link]
- Starkey C. Injuries and illnesses in the national basketball association: A 10-year perspective. Journal of Athletic Training. 2000; 35(2):161-7. [PMID] [PMCID]
- Kofotolis ND, Kellis E, Vlachopoulos SP. Ankle sprain injuries and risk factors in amateur soccer players during a 2-year period. The American Journal of Sports Medicine. 2007; 35(3):458-66.[DOI:10.1177/0363546506294857] [PMID]
- Yde J, Nielsen AB. Sports injuries in adolescents' ball games: Soccer, handball and basketball. British Journal of Sports Medicine. 1990; 24(1):51-4. [DOI:10.1136/bjsm.24.1.51] [PMID] [PMCID]
- Oberg B, Ekstrand J, Möller M, Gillquist J. Muscle strength and flexibility in different positions of soccer players. International Journal of Sports Medicine. 1984; 5(4):213-6. [DOI:10.1055/s-2008-1025908] [PMID]
- Hägglund M, Waldén M, Ekstrand J. Injury incidence and distribution in elite football-A prospective study of the Danish and the Swedish top divisions. Scandinavian Journal of Medicine & Science in Sports. 2005; 15(1):21-8. [DOI:10.1111/j.1600-0838.2004.00395.x] [PMID]
- Waldén M, Hägglund M, Ekstrand J. UEFA champions league study: A prospective study of injuries in professional football during the 2001-2002 season. British Journal of Sports Medicine. 2005; 39(8):542-6. [DOI:10.1136/bjsm.2004.014571] [PMID] [PMCID]
- Duffell LD, Hope N, McGregor AH. Comparison of kinematic and kinetic parameters calculated using a cluster-based model and vicon's plug-in gait. Journal of Engineering in Medicine. 2014; 228(2):206-10. [DOI:10.1177/0954411913518747] [PMID]
- Ishii Y, Hashizume T, Okamoto S, Iwamoto Y, Ishikawa M, Nakashima Y, et al. Cumulative knee adduction moment during jogging causes temporary medial meniscus extrusion in healthy volunteers. Journal of Medical Ultrasonics. 2023; 50(2):229-36. [DOI:10.1007/s10396-023-01288-w] [PMID]
- Youssif RS, Fyaz NA, Hassan KA. Frontal plane projection angle during step down test in subjects with and without patellofemoral pain syndrome. The Medical Journal of Cairo University. 2019; 87:1233-9. [DOI:10.21608/mjcu.2019.53343]
- Herrington L, Myer GD, Munro A. Intra and inter-tester reliability of the tuck jump assessment. Physical Therapy in Sport. 2013; 14(3):152-5. [DOI:10.1016/j.ptsp.2012.05.005] [PMID]
- Gabriel MT, Wong EK, Woo SL, Yagi M, Debski RE. Distribution of in situ forces in the anterior cruciate ligament in response to rotatory loads. Journal of Orthopaedic Research. 2004; 22(1):85-9. [DOI:10.1016/S0736-0266(03)00133-5] [PMID]
- Markovic G, Dizdar D, Jukic I, Cardinale M. Reliability and factorial validity of squat and countermovement jump tests. Journal of Strength and Conditioning Research. 2004; 18(3):551-5. [DOI:10.1519/1533-4287(2004)182.0.CO;2] [PMID]
- Lucci S, Cortes N, Van Lunen B, Ringleb S, Onate J. Knee and hip sagittal and transverse plane changes after two fatigue protocols. Journal of Science and Medicine in Sport. 2011; 14(5):453-9. [DOI:10.1016/j.jsams.2011.05.001] [PMID] [PMCID]
- Sallet P, Perrier D, Ferret JM, Vitelli V, Baverel G. Physiological differences in professional basketball players as a function of playing position and level of play. The Journal of Sports Medicine and Physical Fitness. 2005; 45(3):291-4. [PMID]
- Rismayadi A, Purnamasari I, Novan NA, Firdaus IR, Novian G. Differences in physical conditions for each playing position in basketball athletes. Journal of Physical Education and Sport. 2023; 23(4):844-9. [DOI:10.7752/jpes.2023.04107]
- Delextrat A, Cohen D. Strength, power, speed, and agility of women basketball players according to playing position. Journal of Strength and Conditioning Research. 2009; 23(7):1974-81. [DOI:10.1519/JSC.0b013e3181b86a7e] [PMID]
- Zarei M, Rahnama N, Rajabi R. The effect of soccer players’ positional role in Iran super league on sport injury rates. World Journal of Sport Sciences. 2009; 2(1):60-4. [Link]
- Belali Shamsara G, Rahnama N, Ahzari A. [Investigating the effect of player positions in the Iranian men’s basketball league on the incidence of sports injuries (Persian)]. Sports Medicine and Physical Fitness. 2013; 1(1):33-46. [Link]
- Moreira P, Gentil D, Oliveira Cd. Prevalence of injuries of Brazilian Basketball National Team during 2002 season. Revista Brasileira de Medicina do Esporte. 2003; 9(5):258-62. [DOI:10.1590/S1517-86922003000500002]
- Krosshaug T, Slauterbeck JR, Engebretsen L, Bahr R. Biomechanical analysis of anterior cruciate ligament injury mechanisms: Three-dimensional motion reconstruction from video sequences. Scandinavian Journal of Medicine & Science in Sports. 2007; 17(5):508-19. [DOI:10.1111/j.1600-0838.2006.00558.x] [PMID]
- Perše M, Kristan M, Perš J, Kovačič S. A template-based multi-player action recognition of the basketball game. Paper presented at: Proceedings of the ECCV Workshop on Computer Vision Based Analysis in Sport Environments. 12 May 2006; Graz, Austria. [Link]
- Erčulj F, Supej M. The impact of fatigue on jump shot height and accuracy over a longer shooting distance in basketball. Baltic Journal of Sport and Health Sciences. 2006; 4(63):35-41. [DOI:10.33607/bjshs.v4i63.567]