1. Introduction
During daily activities, soldiers engaged in physical physical activities, including walking and running. These severe physical activities involve potential risks of injury [
1]. It has been reported that about 80%–90% of these injuries are associated with training-related activities [
1]. These injuries often occur in the knee and spine regions [
2]. The risk of injuries in soldiers is 2 to 4 times higher than in the civilian population [
3]. It is reported that lower extremity injuries are common in individuals undergoing military training with incidences of 25% and 82% [
4, 5]. Overuse lower limb injuries include stress fractures, patella-femoral syndrome, and Achilles tendinopathy. During military training [
6], increased running intensity [
7], load carrying [
8], and wearing military combat boots [
9] have all been attributed as causative of different injuries [
10].
The shoe mileage affects the lower limb muscular activities in runners [
11, 12]. Electromyography (EMG) measurements can help identify the different reasons that increased injury risk. Hinz et al. [
13] reported that a softer sole may lead to decreased stress intensity on the metatarsal bones and therefore reduce the possibility of march fractures. Also, EMG activities are associated with running-related injuries [
3]. Previous studies have reported that footwear can have a significant effect on running performance, which in turn can lead to injury. However, the effects of footwear on running mechanics received minimal attention in previous studies, especially in soldiers [
7,
14].
Co-contraction increases energy expenditure leading to a high cost of walking and finally leads to fatigue [
15]. However, insufficient information exists about knee joint muscular contractions when using used and new boots during running. Therefore, this study was conducted to examine the effect of three types of military boots mileage on knee muscular co-contraction during running.
2. Materials and Methods
The type of this study was double-blinded with repeated measures. G*Power software, version 3.1 was used to assess a priori power analysis. The power analysis was performed using the F-test family [
16]. This software showed that at least 15 participants per group are required to achieve large-sized interaction effects. Fifteen healthy individuals (age: 22.3[1.7] years; height: 162.3[6.5] cm; mass: 62.4[9.3] kg) volunteered to participate in this study. All participants were right-footed. Participants had previously worn this shoe-type model. The exclusion criteria included a history of surgery or orthopedic disorders.
Experimental procedures
Participants wore new boots during pre-tests and were measured with used boots during post-test. Before and after the 14 months, mechanical boots testing was used to assess boots’ stiffness. In addition, running mechanics were assessed while running along an 18-m runway at a constant speed. Before starting the study, all participants received three new pairs of military boots (Arsan Sanat Aghanezhad (private company), rubber and polyurethane, and polyurethane thermoplastic made in Iran-Tabriz). The EVA midsole provides stability to provide cushioning. Six months after pre-test, participants performed posttest. Participants were familiarized with the laboratory environment by running 3 times across the walkway. Participants walked at a constant speed during testing. Three true running trials were recorded at both pre and post-test and used for further data analyses. Finally, muscle-specific-maximum voluntary isometric contraction (MVIC) testing was performed to normalize EMG amplitudes.
Experimental setup and data processing
At pre and post-test stages, all participants were asked to run at a constant speed (3.2 m/s) along an 18-m walkway with new boots (Arsan Sanat Aghanezhad (private company), rubber, polyurethane, and polyurethane thermoplastic made in Iran-Tabriz) during pre-tests and used boots during posttest. A wireless EMG system (Biometrics Ltd., Newport, UK) with 8 pairs of Ag/AgCl electrodes (20 mm center-to-center distance; input impedance of 100 MΩ; and common mode rejection ratio (CMRR) of >110 dB) was used to record EMG data of the tibialis anterior (TA), medial gastrocnemius (Gas Med), biceps femoris (BF), semitendinosus (ST), vastus lateralis (VL), vastus medialis (VM), and rectus femoris (RF), and gluteus medius (Glut-Med) muscles of the dominant limb [
17]. The aforementioned muscles were chosen due to their stabilizing role during running [
18]. Raw EMG signals were digitized at a sampling rate of 1000 Hz. According to the surface electroMyoGraphy for the non-invasive assessment of muscles (SENIAM), the skin was cleaned with alcohol [
17]. Maximum voluntary isometric contraction (MVIC) was recorded for each muscle to normalize EMG amplitude. A bandwidth filter of 10–500 Hz and a notch filter of 50 Hz were applied to filter the EMG waves [
19]. The running sub-phases include loading (first 15% of stance), mid-stance (15%–60% of stance), and late stance (last 40% of stance). Two variables were constructed to calculate knee muscular co-contraction, directed co-contraction ratios (DCCR) of agonists and antagonists, and general co-contraction. directed co-contraction ratios (DCCRs) were measured for medial (semitendinosus (SM), vastus medialis (VM), medial gastrocnemius (MG))/lateral (biceps femoris (BF), vastus lateralis (VL)) muscles directed mediolateral knee co-contraction (MLDCCR), medial (VM)/lateral (VL) quadriceps (VM/VLDCCR), and the knee flexors (SM, BF, MG)/extensors (VL, VM) directed knee flexion and extension co-contraction (FEDCCR) [
16]. The DCCRs were measured as follows:
If agonist EMG amplitude>antagonist EMG amplitude;
DCCR=1-antagonist EMG amplitude/agonist EMG amplitude
Else
DCCR=agonist EMG amplitude/antagonist EMG amplitude– 1
Maximum co-contraction is indicated by a DCCR equal to zero, while a minimum co-contraction is indicated by a DCCR of 1 or -1. General co-contraction was measured using the sum of all agonist and antagonist activity.
Statistical analyses
Normal distribution of data was established using the Shapiro-Wilk test. A separate 2 (boots: Rubber vs Polyurethane)×2 (time: Pre vs posttest) analysis of variance (ANOVA) with repeated measures was computed. Effect sizes were determined by partial eta-squared (η2p). The significance level was set at P<0.05. All analyses were performed using SPSS softwarer, version 23.
3. Results
The results showed no significant main effects of “boot” for general knee co-contraction (P>0.05) during all phases. The results showed a significant main effect of “time” for general knee co-contraction during loading response (P=0.030, n2p=0.157). The paired-wise comparison revealed significantly greater general knee co-contraction at the loading response phase in the polyurethane thermoplastic than polyurethane boots. Furthermore, significant boot-by-time interactions were found for general knee co-contraction at push-off phase (P=0.008, n2p=0.302) (
Table 1).
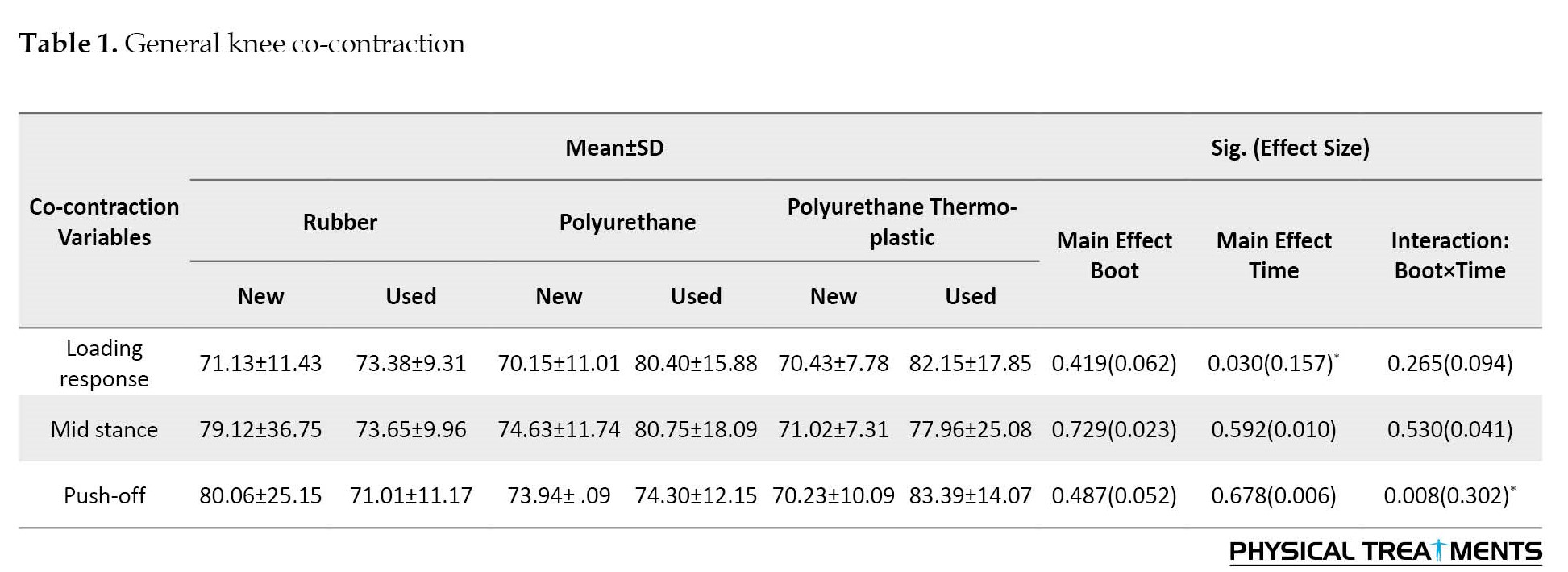
The results showed no significant main effect of “boot”, “time” and boot-by-time for directed vastus lateralis and medialis knee co-contraction knee at all phases (
Table 2).
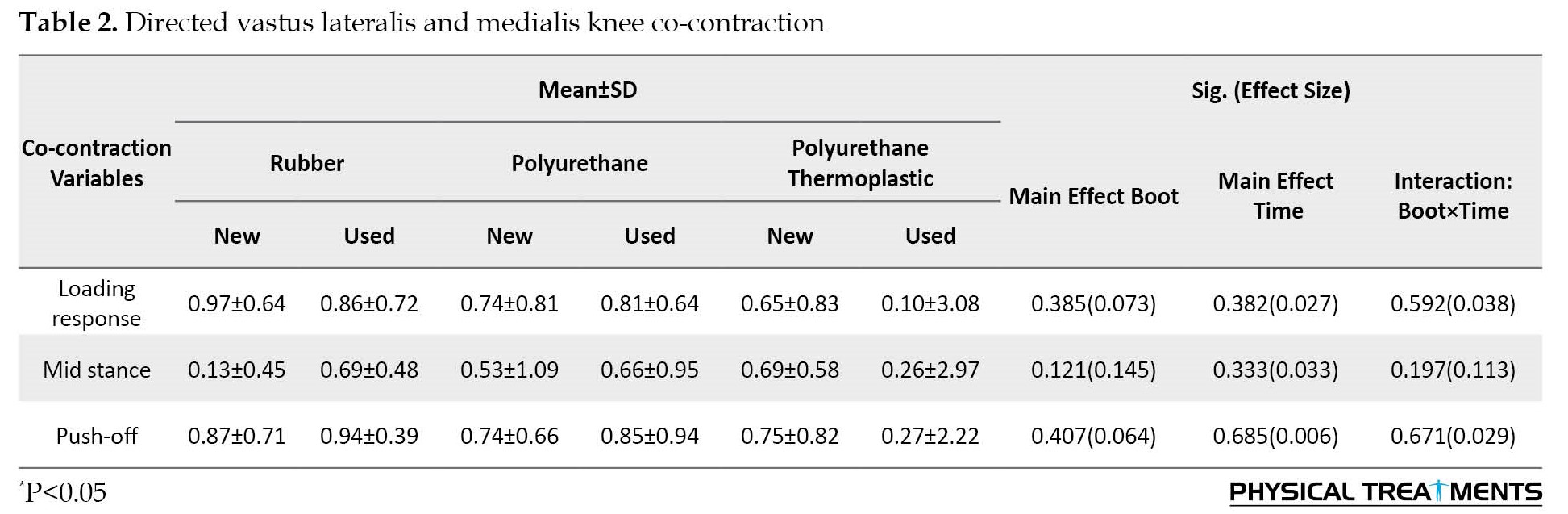
The results demonstrated significant main effects of “boot” for directed knee mediolateral co-contraction (P=0.039, n2p=0.115) during the push-off phase. The results showed a significant main effect of “time” for directed knee mediolateral co-contraction at mid-stance phase (P=0.028, n2p=0.040). The paired-wise comparison revealed significantly greater directed knee mediolateral co-contraction at the mid-stance phase in the polyurethane thermoplastic than the rubber group. The results showed no significant boot-by-time interactions for directed knee mediolateral co-contraction at all phases (
Table 3).
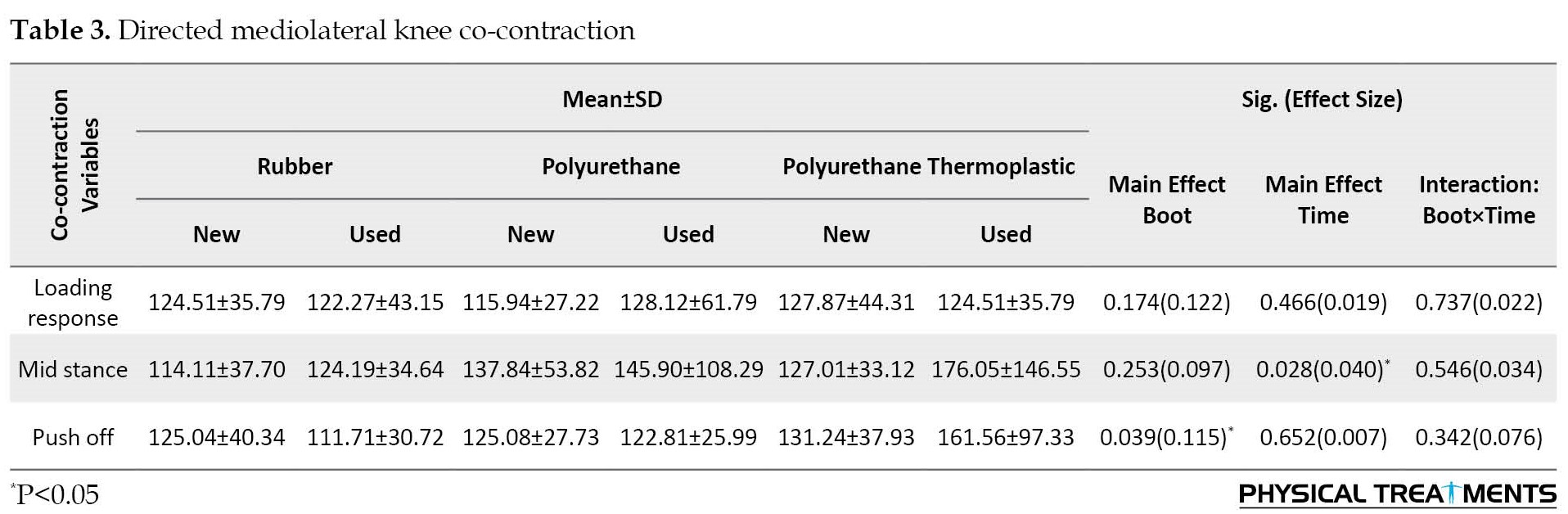
The results showed no significant main effects of “boot” for directed knee flexion and extension co-contraction at all phases (P>0.05). The results showed a significant main effect of “time” for directed knee flexion and extension co-contraction at push-off phase (P=0.017, n2p=0.188). The paired-wise comparison revealed significantly greater directed knee flexion and extension co-contraction at the push-off phase in the polyurethane thermoplastic than that polyurethane boots. Furthermore, significant boot-by-time interactions were found for directed knee flexion and extension co-contraction at the loading response phase (P=0.046, n2p=0.308) (
Table 4).
4. Discussion
This study was conducted to examine the effect of three types of military boots mileage on knee muscular co-contraction during running.
The results revealed significantly greater general knee co-contraction at the loading response phase in the polyurethane thermoplastic than in polyurethane boots. Previous studies have shown that knee joint instability is one of the risk factors for falls [
20]. Increased general knee co-contraction may be associated with instability of the joint after fatigue [
21]. By our results, it can be concluded that knee joint instability while using polyurethane thermoplastic is more than in polyurethane boots.
The results showed no significant group-by-time interactions for directed knee mediolateral co-contraction at all phases. Furthermore, the results revealed significantly greater directed knee flexion and extension co-contraction at the push-off phase in the polyurethane thermoplastic than in polyurethane boots. Hirokawa et al. [
22]reported that hamstring co-contraction has a significant effect on maintaining knee stability, providing synergistic action to the anterior cruciate ligament by preventing excessive anterior displacement and internal rotation of the tibia. Based on our results, it can be concluded that maintaining knee stability in the anterior-posterior direction while using polyurethane thermoplastic is better than in polyurethane boots.
This study has some limitations that should be regarded. Firstly, we did not evaluate the kinematic data. Secondly, we did not evaluate kinetic data, such as ground reaction forces and joint moments. Future studies should assess both kinematic and kinetic data to better establish the effect of military boots mileage on running mechanics.
5. Conclusion
It can be concluded that knee joint instability while using polyurethane thermoplastic is more than using polyurethane boots. Also, our results demonstrated that maintaining knee stability in the anterior-posterior direction while using polyurethane thermoplastic is better than polyurethane boots.
Ethical Considerations
Compliance with ethical guidelines
This study was approved by the Ethics Committee of the Mohaghegh Ardabili University (Code: IR.UMA.REC.1401.026) and was registered at IRCT (IRCT20220714055469N1). All participants signed their written informed consent to participate in the present study.
Funding
This research did not receive any grant from funding agencies in the public, commercial, or non-profit sectors.
Authors' contributions
Conceptualization and writing the original draft: Milad Piran Hamlabadi; Methodology: Milad Piran Hamlabadi and AmirAli Jafarnezhadgero; Investigation: Milad Piran Hamlabadi; Writing–review and editing: Milad Piran Hamlabadi and AmirAli Jafarnezhadgero; Funding acquisition: Iman Bakhshodeh Nia and Hamid Hassannejad; Resources: Iman Bakhshodeh Nia and Hamid Hassannejad; Supervision: Amir Ali Jafarnezhadgero.
Conflict of interest
The authors declared no conflict of interest.
Acknowledgments
The authors thank the participants in this study for their voluntary participation.
References
- Jones BH, Knapik JJ. Physical training and exercise-related injuries. Surveillance, research and injury prevention in military populations. Sports Medicine. 1999; 27(2):111-25. [DOI:10.2165/00007256-199927020-00004] [PMID]
- Jennings BM, Yoder LH, Heiner SL, Loan LA, Bingham MO. Soldiers with musculoskeletal injuries. Journal of Nursing Scholarship. 2008; 40(3):268-74. [DOI:10.1111/j.1547-5069.2008.00237.x] [PMID]
- Kaufman KR, Brodine S, Shaffer R. Military training-related injuries: Surveillance, research, and prevention. American Journal of Preventive Medicine. 2000; 18(3):54-63. [DOI:10.1016/S0749-3797(00)00114-8] [PMID]
- Cowan DN, Bedno SA, Urban N, Yi B, Niebuhr DW. Musculoskeletal injuries among overweight army trainees: Incidence and health care utilization. Occupational Medicine. 2011; 61(4):247-52. [DOI:10.1093/occmed/kqr028] [PMID]
- Swedler DI, Knapik JJ, Williams KW, Grier TL, Jones BH. Risk factors for medical discharge from United States Army basic combat training. Military Medicine. 2011; 176(10):1104-10. [DOI:10.7205/MILMED-D-10-00451] [PMID]
- Davidson PL, Chalmers DJ, Wilson BD, McBride D. Lower limb injuries in New Zealand defence force personnel: Descriptive epidemiology. Australian and New Zealand Journal of Public Health. 2008; 32(2):167-73. [DOI:10.1111/j.1753-6405.2008.00195.x] [PMID]
- Finestone A, Milgrom C. How stress fracture incidence was lowered in the Israeli Army: A 25-yr struggle. 2008; 40(11 Suppl):S623-9. [DOI:10.1249/MSS.0b013e3181892dc2] [PMID]
- Jones BH, Thacker SB, Gilchrist J, Kimsey CD Jr, Sosin DM. Prevention of lower extremity stress fractures in athletes and soldiers: A systematic review. Epidemiologic Reviews. 2002; 24(2):228-47. [DOI:10.1093/epirev/mxf011] [PMID]
- Orr RM, Pope R, Johnston V, Coyle J. Load carriage: Minimising soldier injuries through physical conditioning-A narrative review. Journal of Military and Veterans’ Health. 2010; 18(3):31-8. [Link]
- Andersen KA, Grimshaw PN, Kelso RM, Bentley DJ. Musculoskeletal lower limb injury risk in army populations. Sports Medicine-Open. 2016; 2(1):1-9. [DOI:10.1186/s40798-016-0046-z] [PMID] [PMCID]
- Schulze C, Lindner T, Schulz K, Finze S, Kundt G, Mittelmeier W, et al. The influence in airforce soldiers through wearing certain types of army-issue footwear on muscle activity in the lower extremities. The Open Orthopaedics Journal. 2011; 5:302-6. [DOI:10.2174/1874325001105010302] [PMID] [PMCID]
- Schulze C, Lindner T, Woitge S, Schulz K, Finze S, Mittelmeier W, et al. Influence of footwear and equipment on stride length and range of motion of ankle, knee and hip joint. Acta of Bioengineering and Biomechanics/Wrocław University of Technology. 2014; 16(4):45-51. [PMID]
- Hinz P, Henningsen A, Matthes G, Jäger B, Ekkernkamp A, Rosenbaum D. Analysis of pressure distribution below the metatarsals with different insoles in combat boots of the German Army for prevention of march fractures. 2008; 27(3):535-8. [DOI:10.1016/j.gaitpost.2007.06.005] [PMID]
- Knapik JJ, Graham B, Cobbs J, Thompson D, Steelman R, Jones BH. A prospective investigation of injury incidence and injury risk factors among army recruits in military police training. BMC Musculoskeletal Disorders . 2013; 14:32. [DOI:10.1186/1471-2474-14-32] [PMID] [PMCID]
- Lo J, Lo O-Y, Olson EA, Habtemariam D, Iloputaife I, Gagnon MM, et al. Functional implications of muscle co-contraction during gait in advanced age. Gait & Posture. 2017; 53:110-4. [DOI:10.1016/j.gaitpost.2017.01.010] [PMID] [PMCID]
- Jafarnezhadgero AA, Piran Hamlabadi M, Anvari M, Zago M. Long-term effects of shoe mileage on knee and ankle joints muscle co-contraction during walking in females with genu varus. Gait & Posture. 2021; 89:74-9. [DOI:10.1016/j.gaitpost.2021.07.004] [PMID]
- Hermens HJ, Freriks B, Merletti R, Stegeman D, Blok J, Rau G, et al. European recommendations for surface electromyography. Enschede: Roessingh Research and Development. 1999; 8(2):13-54. [Link]
- Schipplein OD, Andriacchi TP. Interaction between active and passive knee stabilizers during level walking. Journal of Orthopaedic Research: Official Publication of the Orthopaedic Research Society. 1991; 9(1):113-9. [DOI:10.1002/jor.1100090114] [PMID]
- Majlesi M, Azadian E, Farahpour N, Jafarnezhad AA, Rashedi H. Lower limb muscle activity during gait in individuals with hearing loss. Australasian Physical & Engineering Sciences in Medicine. 2017; 40(3):659-65. [DOI:10.1007/s13246-017-0574-y] [PMID]
- Rubenstein LZ. Falls in older people: Epidemiology, risk factors and strategies for prevention. Age and Ageing. 2006; 35(suppl_2):ii37-41. [DOI:10.1093/ageing/afl084] [PMID]
- Esmaeili H, Anbarian M, Hajiloo B, Sanjari MA. [The immediate effect of foot insole on electromyography activity and co-contraction of leg muscles in individuals with flat feet (Persian). Journal of Research in Rehabilitation Sciences. 2013; 9(2):295-307. [Link]
- Hirokawa S, Solomonow M, Luo Z, Lu Y, D'Ambrosia R. Muscular co-contraction and control of knee stability. Journal of Electromyography and Kinesiology. 1991; 1(3):199-208. [DOI:10.1016/1050-6411(91)90035-4] [PMID]